How Much of the 363,397,944m2 Area of Ships’ Submerged Surfaces Arriving to the US Annually is Carrying (Potentially Invasive) Biofouling?
If you place something into the sea anywhere in the world, and leave it there long enough, it is likely to be colonized by marine organisms seeking out a surface to use as a foundation for their adult life. This phenomenon is known as biofouling. Biofouling occurs on surfaces exposed to the air and freshwater too, but it is in the sea that a great diversity and disparity of sessile (attached) animals and seaweed exists, which is why biofouling is both a feature and a bug in the maritime world. In the case of mariners, in particular, it has been a long-time scourge.
Biofouling can deteriorate structures and pose significant challenges to the efficiency of ships. The accumulation of animals and plants on the submerged surfaces of ships increases drag, reduces speed, and diminishes maneuverability. Centuries ago, the impact of biofouling on wooden ships, particularly the activities of burrowing shipworms (Teredo furcifera and other species), could cause the ship’s hull to collapse. This was a fate that befell Christopher Columbus in 1503 when his ships were so compromised that he had to beach them in Jamaica. In the case of modern shipping, biofouling raises fuel consumption and emissions and significantly increases the costs of maritime transport. Fouling can also damage a ship’s engines by clogging the water intake pipes used to cool the engine. For this reason, biofouling remediation, including anti-fouling paints and in-water cleaning technology, is a multi-billion-dollar industry.
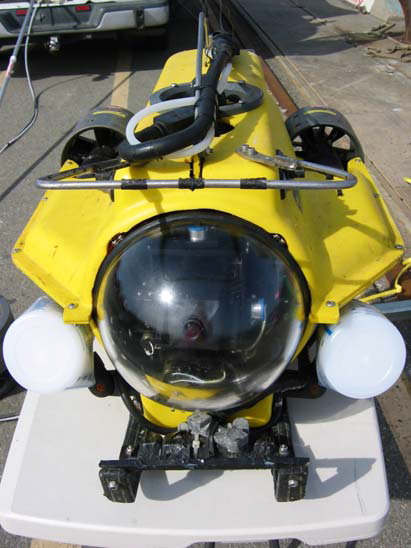
A further consequence of biofouling of ships is its role in re-distributing marine species around the coastlines of the world. Shipping is the dominant vector of nonindigenous species on a world-wide scale, and biofouling is the older partner-vector to ballast water in the overall shipping transfer mechanism. Biofouling organisms attach to ships’ hulls and are transferred to distant geographic areas where spawning, larval release, dislodgement, or a species’ own movements can lead to an introduction event at the recipient port. Ship biofouling is often implicated in the introduction of sessile species – especially those with short larval durations or defenses against harsh voyage conditions – including tunicates, bryozoans, hydroids, barnacles, and mussels. In fact, studies conducted in the marine invasions lab at SERC have highlighted the role of biofouling in driving patterns of invasion across different spatial scales throughout North America1, 2. An estimated 60% of the 257 marine invasions to California’s coast include biofouling as a possible mechanism of initial introduction2.
These deductions about the vectors responsible for invasions are retrospective analyses that use life-history traits of species, timing, and locations of invasions to evaluate the causes of their initial arrival. To gather data on the contemporary vector activity of ships, we must sample the undersides of vessels to determine the prevalence and composition of biofouling communities. This is one of the major research themes for marine ecologists working in the Aquatic Bioinvasion Research & Policy Institute, a partnership between the Smithsonian Environmental Research Center (SERC) and Portland State University (PSU). In addition to in-water sampling of hulls and heterogeneous niche areas of ships (such as rudders, thrusters, and grates), they evaluate shipping patterns, ship dimensions, and species tolerances to voyage conditions to better understand the patterns and processes of contemporary biofouling invasions.
For example, each year an average of 49,382 commercial vessels call on US ports (average number of annual arrivals from 2005 to 2011). The space below the water line of a ship is known as the wetted surface area – this is the space available to marine biofouling - and it can be calculated using ship dimensions and maritime engineering equations4. The cumulative wetted surface area of ships arriving to the United States annually is estimated at 363,397,944m2 or 140 square miles. This area equates to a spatial scale the size of the city of Detroit or the Hawaiian Island of Lanai. Now that’s a lot of hitchhiking space for potential invasive species to settle on. But, how much of that space is actually covered in biofouling and what species occur within those biofouling communities?
The answer (so far) is that biofouling varies greatly among types of ships, types of voyage routes, types of hull maintenance schedules, and several other factors that make this an interesting and challenging question to answer. In 2009, Dr. Ian Davidson (PSU), Dr. Greg Ruiz (SERC) and Christopher Brown (SERC) surveyed the hulls of 22 in-service container ships arriving to the Port of Oakland, California3. The surveys were conducted by divers and remotely operated vehicles (ROVs) whose purpose was to collect biological samples and images at the stern, midship and bow (including hull and niches) of the ships. They found that most ships had less than 1% cover of biofouling on their hull surfaces, but that in combination with niche areas on those same ships, a wide range of organisms were being transferred including green algae, barnacles, hydrozoans, bryozoans, mussels, and tunicates. Some non-native species already established in San Francisco Bay were recorded. The areas of the ships most commonly fouled included rudders, stern tubes and intake gratings. The intake gratings are covers for intake pipes that pump water into the engine for cooling, meaning that biofouling not only carries a risk of invasion, but also has the potential to impact the proper performance of the ship’s engines.
Container vessels like the ones that were surveyed in the Port of Oakland are fast ships that spend a limited time in port, meaning they are likely to be less fouled than a slower vessel that spends more time in port. Following these surveys, the team, including Dr. Davidson, Dr. Ruiz, Dr. Gail Ashton (SERC), and Dr. Chela Zabin (SERC) has been surveying other vessel types in several parts of the country. In the Pacific, cruise ships, barges, general cargo ships, and additional containerships have been surveyed in California, Alaska, Washington, and Micronesia. This summer (2012), the team will also work on the East Coast to sample commercial vessels in Baltimore.
As the number of ships in the data set grows, the team hopes to learn more about the extent, diversity and composition of biofouling - and the causes of their variation - to better understand the biofouling invasion process. In addition, these data are useful for managers looking to evaluate invasion risks and work with shippers to reduce the amount of biofouling being transported by ships. This is particularly relevant in the case of niche areas, because anti-fouling paints focus primarily on reducing biofouling accumulation on hulls of ships because they pay the highest penalties in fuel costs if hulls are fouled. But our research and others’ has shown that niche areas of ships are spaces of concern from an invasion perspective and require attention.
Ultimately, the extent of biofouling on the cumulative hull surface area of ships arriving to the US may be less than 10% and as low as 1%. But 1% of over 350 million square meters is still quite enough space for a huge diversity and abundance of organisms. And the additional heterogeneous nooks-and-crannies of niche areas have been observed as hotspots for biofouling accumulation and transfer. It is little surprise, therefore, that the retrospective invasion analyses for established invaders shows that biofouling remains a primary vector of new invasions throughout the world. Once we have a better understanding of the patterns of biofouling transfers, it will be important to evaluate the critical thresholds that trigger invasions and whether management efforts can be effective at reducing the strength of the vector.
Publications
Ruiz, G., Fofonoff, P., Carlton, J., Wonham, M. & Hines, A. (2000) Invasion of coastal marine communities in North America: apparent patterns, processes, and biases. Annual Review of Ecology and Systematics, 31, 481–531.
Ruiz GM, Fofonoff PW, Steves B, Foss SF, Shiba SN (2011) Marine invasion history and vector analysis of California: a hotspot for western North America Diversity & Distributions. 17: 362-373.
Davidson IC, CW Brown, MD Sytsma, and GM Ruiz. 2009. The role of containerships as transfer mechanisms of marine biofouling species. Biofouling 25: 645–655 dx.doi.org/10.1080/08927010903046268
Davidson I, Sytsma M, Ruiz G (2006) Preliminary investigations of biofouling of ships’ hulls: non-indigenous species investigations in the Columbia River. Report to the US Coast Guard Research & Development Center, Groton, Connecticut. 65pp.